Through the Wall: The Mind-Bending Physics of Quantum Tunneling
- Pushpam Panda
- May 12
- 5 min read
Have you ever thrown a ball at a wall and wished it could just... pass through? In our everyday world, that’s impossible. But in the quantum world, where particles behave in ways that challenge our intuition, such "impossible" events occur frequently. One of these phenomena is Quantum Tunneling, where particles break through barriers that they classically shouldn't be able to cross. Sounds like science fiction? It’s pure science fact.
What Is Quantum Tunneling?
At the scale of atoms and subatomic particles, quantum mechanics rules the roost. Here, particles like electrons behave more like waves than solid objects, meaning they don't have a single, definite position. Instead, their location is described by a "wave function," a mathematical description that shows all the possible places the particle could be.
Unlike our classical understanding where particles travel in straight lines or predictable paths, quantum particles have a probability of being in different places at the same time. This wave-like behavior is governed by the Schrödinger Equation, which predicts the likelihood of finding a particle at any given point.
When a particle encounters a barrier — say, a wall of energy or potential — classical physics says that if the particle doesn’t have enough energy to overcome the barrier, it should simply bounce back. However, in the quantum world, things behave differently. The wave function associated with the particle doesn’t just vanish when it hits the barrier — it penetrates the barrier. There’s a probability that the particle will pass through it, even if it doesn't have the energy to overcome it directly. This is quantum tunneling.

How Does It Work?
To understand tunneling, we need to think about the wave-like nature of particles in quantum mechanics. In simple terms, particles don’t act like little balls but instead exist as waves that spread out over space. These waves can extend beyond obstacles or barriers, even when the particle doesn’t have enough energy to get through by traditional means.
Imagine a ball rolling toward a hill. If the ball doesn’t have enough energy to climb over, it will just roll back. However, if the ball behaves like a quantum wave, part of it could "leak" over the top of the hill, even if it doesn't have the energy to climb it — this is similar to how a quantum particle "leaks" through an energy barrier. This phenomenon occurs due to the probability of the particle's location — it’s possible for the particle to appear on the other side of the barrier, even if classical mechanics would suggest it should stay stuck.
The Math Behind It
The Schrödinger Equation gives us the probability of finding a particle at a certain location. When this equation is applied to a particle encountering a barrier, it shows that the particle’s wave function doesn’t just stop at the barrier but instead decays exponentially as it moves through the barrier. If the barrier is thin enough, the wave function doesn't decay completely, meaning there's still a chance — no matter how small — that the particle will be found on the other side.
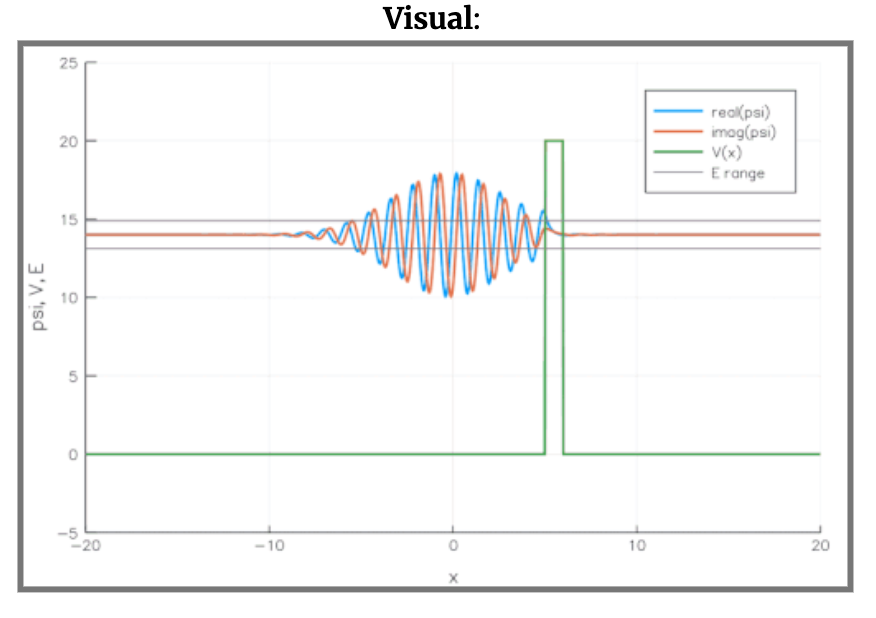
A more detailed animation of a wave function approaching a barrier and decaying.
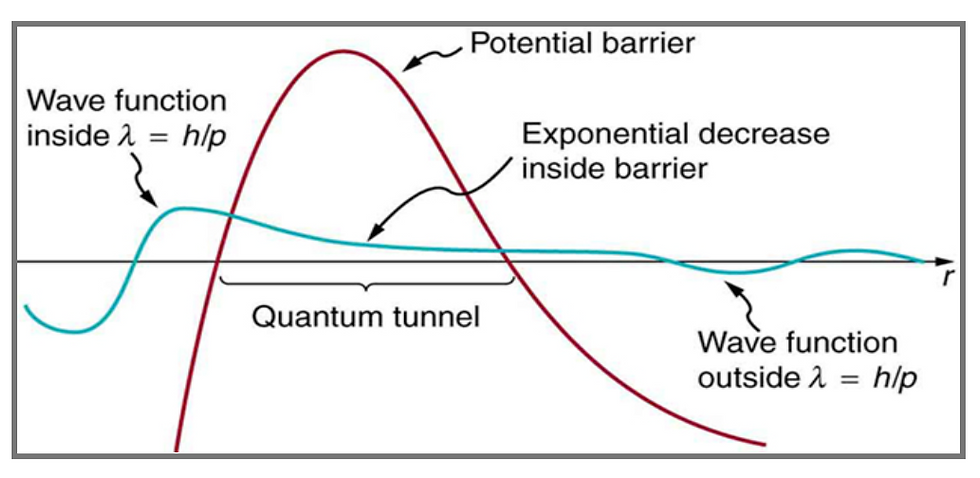
Quantum Tunneling in Technology: A Game-Changer
Now that we understand how tunneling works on a fundamental level, let’s explore the real-world applications of this mind-bending phenomenon.
Semiconductors and Transistors: One of the most significant areas where quantum tunneling is crucial is in semiconductor technology. Transistors, which are the building blocks of modern electronic devices like computers and smartphones, rely on tunneling to operate. As we shrink electronic components to the nanoscale, classical physics no longer applies — particles like electrons use tunneling to flow through transistors even when they don't have the energy to do so by traditional means.As transistors become smaller, the energy barriers that would prevent electrons from passing through shrink as well. When the transistors get to the nanoscale (below 10 nanometers), tunneling becomes more significant, leading to leakage currents. This is why the industry faces challenges in miniaturizing devices further — tunneling can cause energy loss and malfunction.
Scanning Tunneling Microscopes (STM): The STM is a fascinating application of tunneling. This device allows scientists to "see" atoms by measuring the tunneling current between a sharp metal tip and a conducting surface. As the tip moves over the surface, the tunneling current changes in response to the surface’s atomic structure, providing detailed images of individual atoms. The STM was revolutionary, allowing us to directly image the atomic landscape.
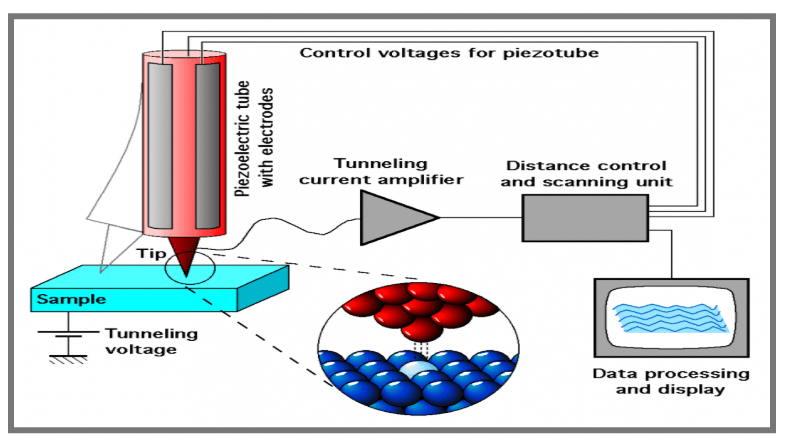
Nuclear Fusion in Stars: Although not a technology on Earth yet, quantum tunneling plays a crucial role in nuclear fusion — the process that powers the sun. For nuclei to fuse, they must overcome their natural electrical repulsion. Normally, they don’t have enough energy to get close enough. However, quantum tunneling allows them to bypass this energy barrier and fuse at lower energies than would be required in classical physics. Without this phenomenon, stars wouldn’t be able to shine!
Quantum Tunneling in the Future: Unlocking the Potential of New Technologies
Quantum Computing: In the world of quantum computing, tunneling is not just a byproduct — it's a feature. Quantum computers rely on the unique properties of quantum bits (qubits) that can be in multiple states at once (superposition). Tunneling allows these qubits to explore multiple possibilities simultaneously, potentially leading to exponential increases in computational power over classical systems. This could revolutionize everything from encryption to drug discovery.
In quantum computers, the process of quantum annealing uses tunneling to help find the optimal solution to a problem by allowing the system to tunnel through energy barriers in the solution space. This allows quantum computers to solve certain problems much faster than traditional computers ever could
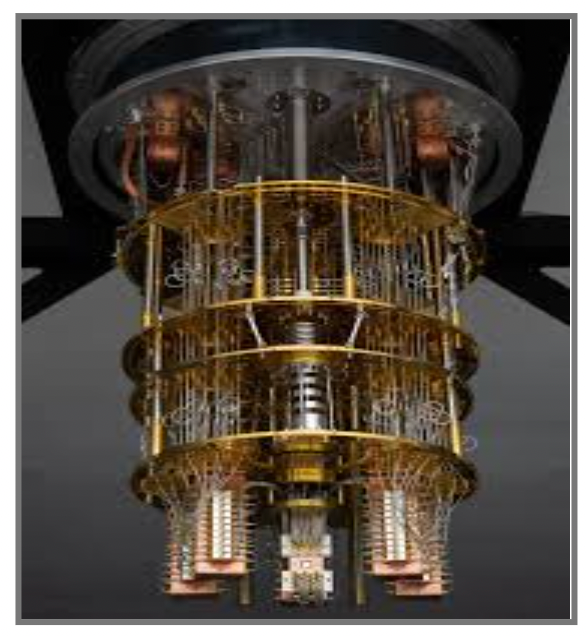
A model of the interior of a quantum computer at the IBM Thomas J. Watson Research Center in Yorktown Heights, N.Y.
Conclusion: The Future Is Unpredictable
Quantum tunneling challenges the boundaries of classical physics. It tells us that the universe behaves radically different at small scales, where the rules we take for granted are more like guidelines. Quantum mechanics reveals a world of probability, not certainty — where particles can be here one moment and there the next, breaking through barriers that seem impassable. As we continue to explore and harness these phenomena, we may just unlock new technologies that could change the world in ways we cannot yet imagine.
Works Cited
Khan Academy (2023). "Introduction to Quantum Tunneling." [online] Available at: https://www.khanacademy.org/science/physics/quantum-physics. This source offers an accessible introduction to quantum mechanics and the concept of tunneling.
Energy.gov (2023). "Quantum Mechanics: How Tunneling Plays a Role in Fusion." [online] Available at: https://www.energy.gov/science/doe-explainsquantum-mechanics. This provides insights into the role of quantum tunneling in nuclear fusion.
Britannica (2023). "Quantum Tunneling." [online] Available at: https://www.britannica.com/science/quantum-tunneling. An authoritative explanation of quantum tunneling and its applications.
Cohen-Tannoudji, C., Diu, B., & Laloë, F. (2021). Quantum Mechanics: A Modern Development. New York: World Scientific. A foundational text in quantum mechanics that covers tunneling and many other phenomena in-depth.
Wikipedia (2023). "Scanning Tunneling Microscope." [online] Available at: https://en.wikipedia.org/wiki/Scanning_tunneling_microscope.
Comments